The Deepest Grave: The Science of Nuclear Waste Disposal
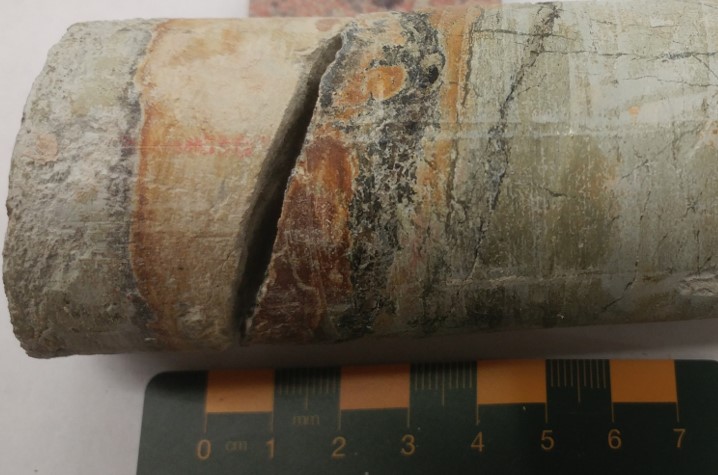
On November 28, 2024, Canada made a historic decision: the Nuclear Waste Management Organization (NWMO) selected a piece of rocky wilderness near the town of Ignace, Ontario, as the location where 100 000’s tonnes of almost inconceivably toxic nuclear waste will be buried for millions of years. The idea is controversial, to say the least. Indeed, the controversy often gets in the way of a discussion of the science of how this actually works.
What is Nuclear Waste?
Radioactive waste products are created mainly by nuclear energy but also some areas of medicine, science, and other industries. It’s classified as low, intermediate, or high level based on how intense the radioactivity is and how long it lasts. Low and intermediate level waste makes up the majority of nuclear waste but poses little long-term safety risk and can generally be easily disposed of. High-level waste is a different beast altogether.
High-level nuclear waste is the remains of nuclear fuel which has been ‘burned’ for energy in a nuclear reactor. This Used Nuclear Fuel (UNF) is so radioactive it produces enough heat to melt itself; this is prevented by storing it underwater. The radioactivity of UNF decreases exponentially with time as the most unstable isotopes decay out of existence. After about ten years the danger of meltdown has passed, and it can be removed from underwater storage and repacked into large concrete and steel silos near the reactor site for interim storage. So far, this is as far as we’ve gotten. Canada has accumulated about 60 000 tonnes of UNF since the 1960’s, with more coming every year.
Although UNF is constantly becoming less radioactive, it will never cease emitting radiation entirely. It takes about 1-10 million years to return to the radioactivity of the natural uranium ore it was made from. These ores don’t cause harm to the environment, therefore UNF disposal plans must be designed for timescales of at least 1 000 000 years. For context, our species has existed for about 300 000 years, the oldest known cave paintings are about 64 000 years old, and the Great Pyramid of Giza is about 4600 years old.
A graph of how the radioactivity of UNF decreases over time. The exact amount of time required to return to the radioactivity of natural ores depends on the grade of the ore considered. The inflection point in the chart is due to the relatively rapid decay of intensely radioactive fission products.
What are we going to do with it?
Many disposal concepts ranging from the ingenious (i.e. recycling it into new nuclear fuel) to the insane (like shooting it into the sun) have been proposed over the years, but today there is a near universal consensus that the best option is deep burial in a Deep Geological Repository (DGR). Canada’s plan, which is similar to the plans of most other nations with UNF, calls for UNF to be entombed at least 500 m underground in a body of suitably stable bedrock for over 1 million years.
The DGR concept ensures safety with a defense-in-depth strategy, with multiple layers of independent engineered and natural barriers between the UNF and the wider world.
The waste itself consists of pellets of uranium oxide sealed inside highly corrosion-resistant Zircalloy tubes. Firewood-sized bundles of these tubes are sealed in thick carbon steel canisters clad with a layer of corrosion-resistant copper; these canisters are then packed in large blocks of highly compacted bentonite clay. These blocks would be placed into a system of specially constructed tunnels, with any remaining space backfilled with more bentonite.
Diagram of the layered barriers in a DGR.
Now that a site has been chosen, the next phase will be characterizing the site with detailed geologic studies and going through the process of seeking regulatory approval from the Canadian Nuclear Safety Commission. This is expected to take about 10 years. If the plans are approved construction, which is expected to take another 10 years, will begin.
Once complete the repository will receive UNF for around 40 years. After this the repository will enter a period of extended monitoring lasting several decades before being sealed for good. It is only at this point that we’re truly committed to leaving the UNF where it lies. Once the tunnels and access points are filled with bentonite, sand, asphalt, and concrete the surface buildings will be demolished and the area returned to nature. If all goes to plan there will be no trace besides written records that the DGR and its contents ever existed.
In Canada planning, building, operating, and paying for all of this is the responsibility of the NWMO, an independent non-profit the nuclear industry was mandated to create and fund.
Why this plan?
In order to cause harm to people or the environment, some of this used nuclear fuel would have to escape the DGR and make its way back to the surface. Putting aside volcanic eruptions, which only occur in known areas which are easily avoided, the only realistic way this could happen is through movement of groundwater.
The role of geology in selecting the natural barriers (the host rock) is obvious. The rock surrounding the DGR must be stable and highly impervious to water. The Ignace site is located in granite, which is extremely waterproof, as long as it remains unfractured, as well as being a very durable building material. The region last experienced geologic activity, like earthquakes or volcanoes, over 2 billion years ago. Being in the middle of a stable continent, it’s highly unlikely to ever experience them again.
Depth is an important factor, building deeper would theoretically increase protection but would also increase the difficulty of construction; the proposed 500–800-meter depth provides a good compromise between these competing factors. It’s also a common depth for underground mines, something Canadian engineers have extensive experience with.
The bentonite sealant is a ‘swelling’ clay. When it comes into contact with water it absorbs a small amount and expands, sealing the gap the water came through. Not only is it one of the best waterproofing materials known, but being a natural clay, it’s known to be stable over millions of years.
The steel canister is designed to resist crushing in rockfalls or earthquakes but is prone to corrosion; its copper cladding is armor against this. Since copper naturally occurs in its metallic (native) state and has been worked since ancient times we know how it resists corrosion underground over thousands and millions of years.
Many natural materials are used in DGR designs (i.e. copper and clay vs stainless steel and silicone) because we know how they behave over millions of years.
Even the UNF itself is a synthetic version of the natural mineral uraninite. These substances have the same chemical formula (UO2), differing only in the impurities; in uraninite these are mainly harmless calcium, silicon, and rare earth elements, whereas the impurities in UNF, fission fragments and actinides formed by nuclear reactions, produce the overwhelming majority of UNF’s radioactivity.
A) a crystal of natural uraninite. B) Uranium oxide nuclear fuel pellets.
The behavior of these materials is easy to predict over the ‘short’ term, but uncertainties mount over 10 000’s – 100 000’s of years and detailed engineering studies become less useful. Even the most well-made materials will eventually degrade. What happens beyond this point is increasingly controlled by the Earth itself.
What does the Science say?
One of the main arguments against geologic disposal is that it has never been successfully done before. Not only is this argument defeatist (imagine if the Wright brothers had said ‘no one’s invented the airplane before, therefore it’s not possible and let’s not try’), it’s not really true either. The oldest buried UNF has existed for nearly 2 billion years.
Thanks to a unique set of circumstances which couldn’t be repeated today 14 uranium ore bodies at Oklo, Gabon sustained fission chain reactions 1.95 billion years ago. This transformed the uraninite ore into natural UNF, which was already buried in the bedrock; a natural analogue for a manmade DGR.
The UNF at Oklo formed in shallow, fractured, porous sandstone which was saturated in flowing groundwater. Obviously, the engineered barriers, i.e. canister and bentonite, were never there to start with. The Oklo DGR analogue might as well have been designed to fail…and yet it didn’t. Years of intensive study reveal that essentially all of the UNF has remained exactly where it formed. Again, that’s over nearly 2 billion years, about 2000 times longer than we need a DGR to last.
Oklo is unique in having formed UNF, but not in remaining stable over millions to billions of years. Most uranium deposits contain uraninite, therefore nature has provided us with hundreds of examples of how we should expect UNF to behave under all kinds of circumstances.
The Cigar Lake deposit in northern Saskatchewan, Canada, for instance, has lasted for 1.6 billion years despite being located in porous, leaky rocks. There is no trace of the deposit or its radioactivity at the surface. In fact radioactive ores located at repository depth contain their radioactivity so well that they are notoriously difficult to find: companies routinely come up empty despite spending many years and millions of dollars searching.
Geologic studies have helped establish principles for nuclear waste disposal. These include construction in seismically stable regions lacking in conceivably exploitable underground resources (which could lead to accidental breaches during future mining), and the use of purely passive (not requiring any active human or machine involvement) and naturally stable safety measures.
The real key, however, is oxygen. This highly reactive gas is destructive to many metals and geologic materials, especially uraninite and UNF. Fortunately, most deep geologic formations and any contained groundwaters contain essentially no free oxygen. As long as a DGR is located in such an environment the materials within are inherently chemically stable and are remarkably difficult to move.
Today, much of the science of nuclear waste disposal is focused on worst-case scenarios. My work (the geochemistry of natural analogues for DGRs is the focus of my PhD program) looks at the Kiggavik uranium deposits, which are located in deeply fractured rocks intensely affected by glaciation. Here the weight of former icesheets appears to have forced oxygen-rich water to flow right into the heart of the uranium ore over 100 000’s of years. Even under these near worst-case conditions the uranium has moved only a few centimeters. The illite clay, with a little help from a few other minerals, appears to have acted as a filter, stopping uranium and its radioactive decay products from escaping.
Uraninite (black material) in drill core from the Kiggavik deposit. The large vein on the right formed ~1.55 billion years ago, while the small feature to the left marks the furthest extent of uranium movement caused by water moving down the large open fracture. The uranium took 100 000’s of years to move through the clay in the host rock.
We can’t guarantee a DGR will never cause harm for the same reason I can’t guarantee I will never be struck by lightning: there are limits to human knowledge. It’s notoriously difficult to prove something will never happen. What we can say is that the mountain of evidence we’ve accumulated says there’s no reason to expect deeply buried nuclear waste to do anything but sit there for many millions of years. Failure would require not only the complete failure of human science and engineering, but the Earth itself would have to behave in a way that’s so out of character that we’re hard pressed to find a single example of it happening anywhere, ever.
A Long Legacy
Ultimately the biggest unknown is future humans.
Some have raised concerns that UNF may eventually be removed from a DGR and weaponized. This is theoretically possible, but one must keep in mind the difficult, time-consuming, expensive, and obvious nature of blasting your way through 100’s of meters of rock; anyone having the resources to do so would presumably have access to much easier ways of causing trouble.
Although the location of a DGR will be recorded, there is a chance that it may eventually be lost to history. If someone were to somehow accidentally unearth a DGR in say 100 years, the radioactivity of the UNF will be 99.99% lower than when it was produced, and they would presumably have far more advanced technology to detect and manage the hazard than we do today. Indeed, it may one day be viewed as a resource, much as Oklo’s natural UNF was mined to be made into modern nuclear fuel.
The UNF will exist for millions of years no matter what we choose to do with it, we’re probably better off leaving it somewhere our descendants will only have to deal with it if they choose to.
Assuming the Ignace DGR gets approved and built, and that’s far from guaranteed, Canada’s UNF won’t have a permanent home for at least 2 more decades. The good news is that there is no looming nuclear waste crisis on the horizon. Unlike greenhouse gases, microplastics, or many other forms of pollution, nuclear waste is a relatively small amount of solid material which isn’t prone to becoming dispersed in a way we can’t control. There’s a reason there’s never been a serious accident involving UNF during storage or transportation.
Estimated radiation doses experienced by people obtaining drinking water from a deep well directly above a DGR under various scenarios. Even assuming multiple barrier failures the dose is less than 1% of the natural background radiation exposure.
In principle we could continue doing what we’ve been doing for decades, perhaps even forever. There are some who advocate for this, but why? Why kick a can down the road forever when we have everything we need to dispose of it permanently?
Takeaways
After many years of planning and consulting Canada is moving forward with plans to bury its nuclear waste in a deep geologic repository. Far from being the unprecedented feat of engineering it’s often portrayed as, it’s actually an attempt to recreate common and well understood natural processes. The necessary science, technology, and financing are in place, now the question is whether we have the will to implement them.