Supercontinent Cycles: Geology on the Largest Scale
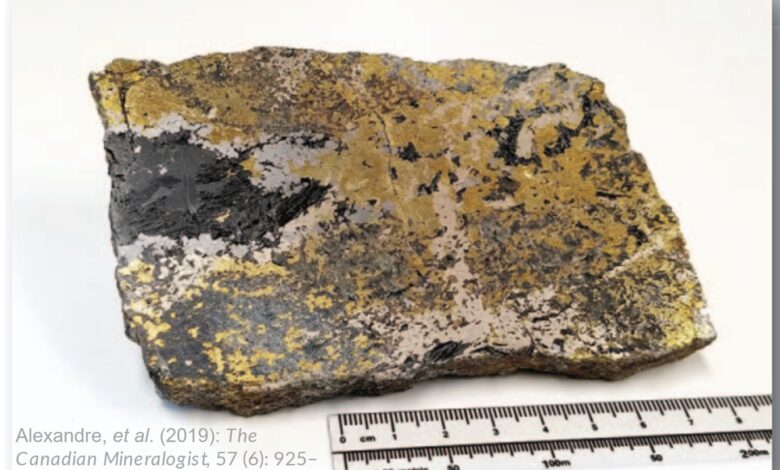
Geology can be hard to predict on the local scale (there’d be a lot fewer jobs for geologists otherwise) but on larger scales there is an order to how and when mountains, mineral deposits, and more occur. The theory of plate tectonics explains most geologic features, but even tectonics have changed throughout geologic time, with supercontinents assembling, stabilizing, and breaking up in cycles spanning 100’s of millions of years. These cycles have shaped not only our mineral resources, but the entire world.
Introduction
Today, the world is divided into continents separated by multiple oceans, but this wasn’t always the case. You’ve probably heard of Pangea, the vast collection of (nearly) all the world’s landmass which was home to the dinosaurs from ~336-175 million years ago. Pangea, however, was not the first supercontinent. Decades of painstaking research has revealed the existence of at least 3 previous supercontinents dating back over 1.5 billion years. While the continents themselves are long gone, traces of them still linger in rocks and mineral deposits around the world.
Illustration of how upwelling mantle creates oceanic crust and seafloor spreading at mid-ocean ridges, with downwelling and subduction under continents.
Supercontinent cycles are driven by the same force as plate tectonics: mantle convection. Heat from the core causes material in the mantle to slowly rise, where it comes to the surface we get volcanoes which create new oceanic crust. The creation of this new seafloor spreads the ocean basins apart, pushing the continents along with them. Eventually, mantle material near the surface cools enough that it sinks back towards the core, creating an area of downwelling. Continents are too buoyant to sink, they float on the dense mantle like unsinkable ships, but they are not completely unaffected. Hot, young oceanic crust tends to push continents away from centers of upwelling and towards areas of downwelling. As continents move cold, old oceanic crust gets forced underneath continents (subduction), creating long belts and arcs of mountains and volcanoes as the subducted crust heats up and melts. When continents collide they stick together forming large mountain ranges and, sometimes, supercontinents.
Supercontinent basics
Supercontinents are loosely defined as an amalgamation of at least 75% of the world’s land. Their lifecycles are divided into three phases: assembly, stability, and dispersal.
During the assembly stage smaller continents drift towards each other and eventually collide to form a single landmass. This phase is dominated by seafloor spreading and internal orogenies, where continents collide with each other to produce massive mountain ranges in the interiors of newly formed continents. This is how the Himalayas were created.
The stability phase begins once most of the landmass has assembled. Subduction of oceanic crust continues under the continental margins, producing smaller mountain belts and long volcanic chains along the periphery of continents similar to the Rocky Mountains. Mountain ranges formed during assembly slowly erode, producing vast amounts of sediment that form equally vast sedimentary basins covering large swaths of the interior.
The dispersal phase begins as the supercontinent breaks up (rifts) into smaller continents which disperse around the globe. It’s thought that supercontinents are large enough to trap heat from the mantle below which builds up over millions of years. Eventually this heat triggers melting and volcanism on such a scale that the land is cracked open and seafloor spreading begins, creating a new ocean basin and forcing the continents apart. Dispersal is associated with large amounts of volcanism, mainly in the form of seafloor spreading in new ocean basins and volcanic arcs along continental margins.
How do we know?
Reconstructing the last supercontinent is as simple as puzzle-piecing the continents back together. Earlier supercontinents have been reconstructed based largely on correlating rock formations between areas and paleomagnetic data. When magnetic minerals form they tend to be aligned with the Earth’s magnetic field, recording a snapshot of it at the time. With enough data you can work out the approximate positions of where these rocks formed relative to the Earth’s magnetic poles. Reconstructing early supercontinents is a complicated and painstaking process, and the layout, and even existence, of many is controversial.
How Supercontinents Control Ore Deposits
Illustration of how the frequency of deposit types changed over the life of the Nuna supercontinent.
The early phases of assembly involve large amounts of seafloor spreading, which favors the formation of mineral deposits related to spreading such as volcanogenic massive sulfides (VMS), while the later collision phases produce mountain chains and favors deposits such as orogenic (lode) gold. Assembly is also a period of heightened volcanic activity, which is linked to many deposit types such as porphyry copper, Iron-Oxide-Copper-Gold (IOCG), magmatic nickel-copper-platinum group element, and epithermal gold. In order for us to mine these deposits, however, they must be preserved into modern times. VMS deposits, which form in subduction-prone oceanic crust, are particularly vulnerable to being destroyed, however they are more likely to be preserved if they are caught up in the closure of ocean basins during supercontinent assembly. The assembly phase often stands out as a time of particularly rich mineral deposits.
A stable supercontinent environment favors deposits which require deep sedimentary basins such as Mississippi Valley type lead-zinc, sediment-hosted copper and unconformity uranium. It’s not uncommon for supercontinents to rift slightly without fully breaking up; these periods favour rare types of volcanism which produce some IOCGs and rare earth element-bearing carbonatite deposits. Although deposits such as VMS still form, they are unlikely to be preserved without continental collisions to trap them. Erosion of mountain ranges may also destroy some of the deposits formed during assembly. On the whole, stability tends to be a relatively sparse period when it comes to mineral deposits.
The dispersal phase has a great deal of volcanism in the form of seafloor spreading and volcanic arcs, which is favorable for deposits such as VMS, epithermal gold, and porphyry copper. The high rates of erosion and subduction and lack of continental collisions during these periods, however, means that relatively few of these deposits survive. Eventually, the dispersed continents begin to collide again, and the supercontinent cycle begins again.
Supercontinents also shape the global climate. With most of the landmass far from the ocean the interiors of supercontinents tend to create a lot of desert. When too much land becomes concentrated near the poles it allows large ice sheets, which need solid land beneath them, to form, potentially triggering global ice ages. Changes in the amount of volcanic activity, erosion, and seafloor spreading due to supercontinent cycles also affect the chemistry of the atmosphere and oceans in many ways.
A Quick Tour of the Supercontinents
A reconstruction of Pangea, with modern continents superimposed.
The most recent supercontinent was Pangea, which existed between 336-175 million years ago. Notable deposits of Pangea include lead-zinc deposits of the Mississippi Valley, USA, formed during the stability phase, and most of the world’s porphyry copper, which formed along continental margins during dispersal, which is still ongoing today. Pangea also shaped the dinosaurs. They first emerged about 230 million years ago, near the maximum of Pangea’s size. The vast desert of Pangea’s interior is thought to have been too dry for dinosaurs, however they were able to expand their range and diversity significantly as Pangea began to break up and deserts gave way to lusher terrain. The large amount of CO2 produced by rift-related volcanoes also kept the global temperature high enough for reptiles to spread to high latitudes. Ultimately this volcanism may have been too much of a good thing: volcanic gases are now thought to have built up and poisoned the atmosphere, eventually killing off many dinosaurs. The asteroid was only the final straw.
Pannotia (633-573 million years ago) was rather short-lived. In fact, whether it existed at all is has been hotly debated in recent years. It’s break up, assuming it existed, may have triggered changes to ocean chemistry and sea levels which led to the Cambrian explosion, the rapid emergence of new species which set the stage for complex life on Earth.
Rodinia (1130-750 million years ago) is something of an oddball. Dates for its formation and breakup vary widely from source to source, although it is generally accepted to include much of the ‘boring billion’ (1.8-0.8 billion years ago). During this period surprisingly few rocks and mineral deposits are known to have formed. It’s thought that Rodina is so boring because it formed from resembling a few large pieces of the last supercontinent, which meant less newly formed land and deposits overall. These pieces also came together in such a way that few of the new deposits ended up in the interior where they were likely to be preserved.
Nuna (AKA Columbia; 1820-1350 million years ago) makes up for its’ successor’s boringness. Many of the world’s richest orogenic gold and VMS districts formed during its’ assembly around 1800 million years ago. Its’ stability was also associated with world-class IOCG mineralization, such as Olympic Dam, Australia, and unconformity uranium deposits in Canada’s Athabasca Basin. Nuna appears to have had a complex history, with many collisions during assembly followed by multiple failed rifts. This rich history may explain its unusual endowment of metals.
Copper-zinc ore from a VMS deposit in the Flin-Flon area, Canada, formed during assembly of Nuna.
Older supercontinents may have existed, but it’s difficult to be sure as the rock record becomes thinner as we go back in time. Plate tectonics may also have operated differently early in Earth’s history due to the planet having a hotter mantle back then.
Summary
Supercontinent cycles have shaped our world for at least 1.8 billion years, and possibly much longer. While these cycles may seem to have little to do with our modern lives, they have shaped the ground under our feet, the metals we build our civilizations with, and the history of life itself.
- Pehrsson, S. J., Eglington, B. M., Evans, D. A. D., Huston, D., and Reddy, S. M. (2016): Metallogeny and its link to orogenic style during the Nuna supercontinent cycle. In: Li, Z. X., Evans, D. A. D. and Murphy, J. B. (eds) 2016. Supercontinent Cycles Through Earth History. Geological Society, London, Special Publications, 424, 83– 94.https://www.researchgate.net/publication/279159955_Metallogeny_and_its_link_to_orogenic_style_during_the_Nuna_supercontinent_cycle (academic article)